AURA Ventilator
Engineering Resilience in a Global Crisis
2020-21
Team
Corey Mack (Technical Program Manager, Product Design Engineer, and Industrial Designer), Ben Parnas & Andrew Kowalczyk (Electronics & PCB design), Julian Chiesa (UI/UX), Mahfuza Ahmedin (Regulatory, Quality, & Compliance), Juan Jackson (Project Management)
Project Motivation
Aura Ventilator emerged from a simple, urgent need during the COVID-19 pandemic: to create a life-saving ventilator that could be built quickly and affordably when existing stockpiles were exhausted, and supply chains were severely strained. I led the design and development of this adaptive, low-cost ventilator, focusing on efficiency, manufacturability, and real-time adaptability. This project combined deep technical knowledge with strategic decision-making, delivering a scalable solution when it was needed most.
Concept Overview
The Aura Ventilator was developed to address the critical shortage of ventilators during the COVID-19 crisis. Recognizing that production delays could cost lives, I focused on delivering a robust, adaptable design that could be manufactured domestically using readily available materials.
Iteration and Adaptation: A Journey of Redesign
From concept to final prototype, the Aura Ventilator underwent multiple iterations, each informed by rigorous testing and real-world constraints. This iterative approach ensured that the final product met the highest performance, safety, and manufacturability standards.
Challenges of the Initial Design:
• Inconsistent Flow Rates: The initial reciprocating pump design caused a near-sinusoidal variation in the volumetric flow rate, making it difficult to predict the exact volume delivered. This affected the system's reliability, especially for patients with varying respiratory needs.
• Volume Measurement Errors: The early design relied on step counting to estimate displacement, leading to miscounts when pressure changes or mechanical variances caused the bellows to miss its full range of motion.
• Limited Sensor Feedback: To cut costs, we used only a few pressure sensors, leading to false alarms and inaccuracies. The ventilator’s performance suffered in non-standard conditions like variable altitudes and temperatures.
• Safety Risks During Power Loss: Without passive airflow mechanisms, the ventilator posed a risk of cutting off a patient’s air supply during a power failure.
Strategic Redesign:
• Enhanced Flow Control: Switching to a lead screw mechanism for linear actuation solved the issues with volumetric flow variability. Coupled with a mass flow rate sensor, this provided precise volume delivery, eliminating the unpredictability of the original design.
• Robust Sensor Integration: Adding mass airflow sensors and an optical limit switch created a closed feedback loop, allowing the ventilator to self-correct and adjust output dynamically. This improved accuracy, reduced false alarms, and expanded the device's ability to function under varying environmental conditions.
• Improved Safety Features: Integrated normally open solenoid valves allowed patients to continue breathing if power failed, while strategically placed ventilation holes ensured airflow even if the unit was knocked over, maintaining safety in critical situations.
• Low-Pressure Pneumatics: A shift to low-pressure tubing reduced resistance and allowed the use of more flexible materials, improving compatibility with various oxygen sources and minimizing supply chain issues.
Key Innovations & Design Improvements
• User-Centric Interface: I specified an OLED display for its high contrast and clarity, ensuring that critical metrics were visible even in low-light conditions. I also designed the GUI to focus on intuitive controls, reducing training needs and minimizing potential user errors.
• Modular & Lightweight Design: The ventilator’s modular build allowed for rapid assembly with just 20 bolts, making it easy to repair and upgrade. This design reduced manufacturing costs and enabled swift deployment in emergency settings.
• Adaptive Control Logic: Using flow sensors and a homing sensor for the bellows, the ventilator reset its cycle with each stroke, minimizing cumulative errors and ensuring consistent performance. This adaptive control system adjusted to patient needs in real-time, making it a versatile solution in varied clinical environments.
Efficient Development with 3D Printing
During development, I leveraged 3D printing to accelerate testing and validation. This approach allowed us to quickly prototype parts and test them for assembly and fit, bypassing the delays associated with traditional manufacturing processes. The ability to evaluate components in physical form, rather than relying solely on digital CAD models, helped us iterate rapidly and refine the design before committing to production tooling.
Strategic Manufacturing Decisions
When it came time for full-scale production, I implemented several strategies to keep costs down and ensure flexibility during a time of widespread logistical challenges:
• Tooling Strategy for Display Enclosure: To navigate supply chain disruptions, I specified two different display heights with the same width for the final build. This allowed us to switch between display models seamlessly if one became unavailable, ensuring continuous production. The fascia that housed the displays used the same rear mold but had unique front molds for each display size, reducing tooling costs by 25%.
• Clever Use of Living Hinges: By incorporating living hinges into the design, I eliminated the need for more complex molds with side pulls. This reduced tooling complexity and costs while maintaining durability in the ventilator's structure.
• Part Consolidation: Wherever possible, I combined components or eliminated unnecessary parts, simplifying the overall assembly and reducing material usage. This approach not only cut costs but also made the ventilator easier to produce at scale.
Impact
• Critical Funding Support: The project was supported by a grant from the Schmitt Foundation, alongside contributions from Autodesk, the Morning Bird Foundation, Protolabs, and the University of Houston Victoria. This funding enabled the rapid prototyping and development of a scalable solution.
• Cost-Efficient Manufacturing: Strategic decisions in tooling and sourcing allowed the ventilator to be produced at 40% of the cost of traditional models, while maintaining flexibility to adapt to supply chain challenges.
• Expanding the Performance Envelope: By addressing initial design flaws, I increased the ventilator's range of use, making it suitable for a wider variety of patients, including those with higher oxygen needs and different lung capacities. This adaptability made the Aura Ventilator an essential tool in diverse healthcare environments, from ICUs to makeshift field hospitals.
• Cost-Efficient Manufacturing: Strategic decisions in tooling and sourcing allowed the ventilator to be produced at 40% of the cost of traditional models, while maintaining flexibility to adapt to supply chain challenges.
• Expanding the Performance Envelope: By addressing initial design flaws, I increased the ventilator's range of use, making it suitable for a wider variety of patients, including those with higher oxygen needs and different lung capacities. This adaptability made the Aura Ventilator an essential tool in diverse healthcare environments, from ICUs to makeshift field hospitals.
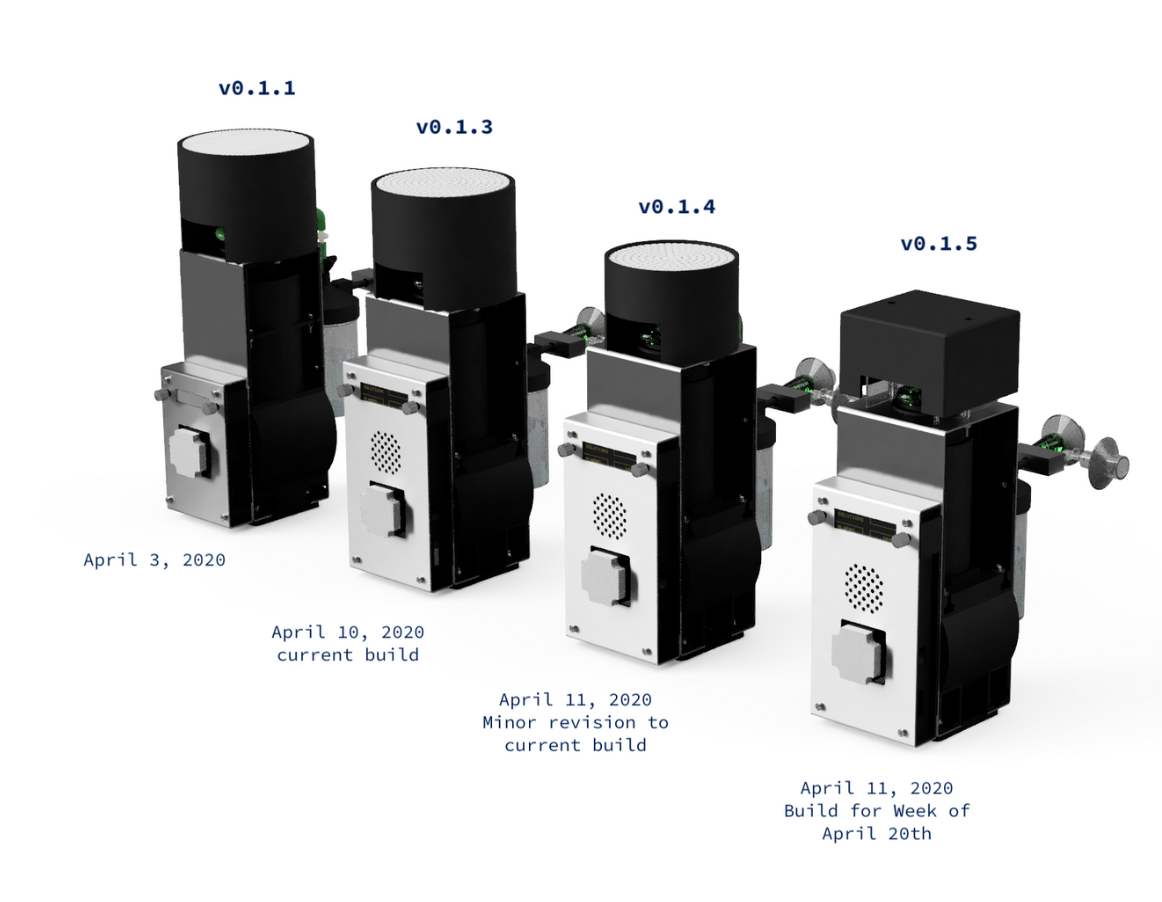
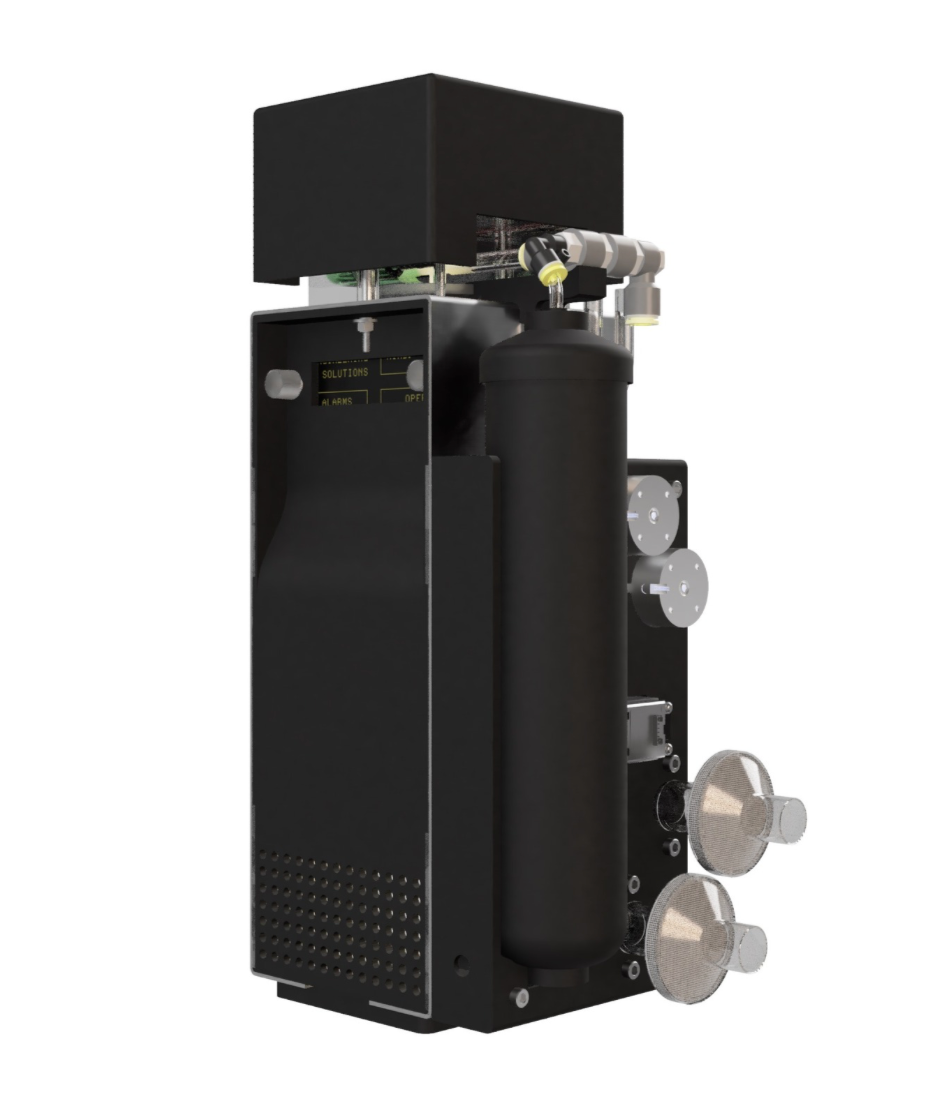
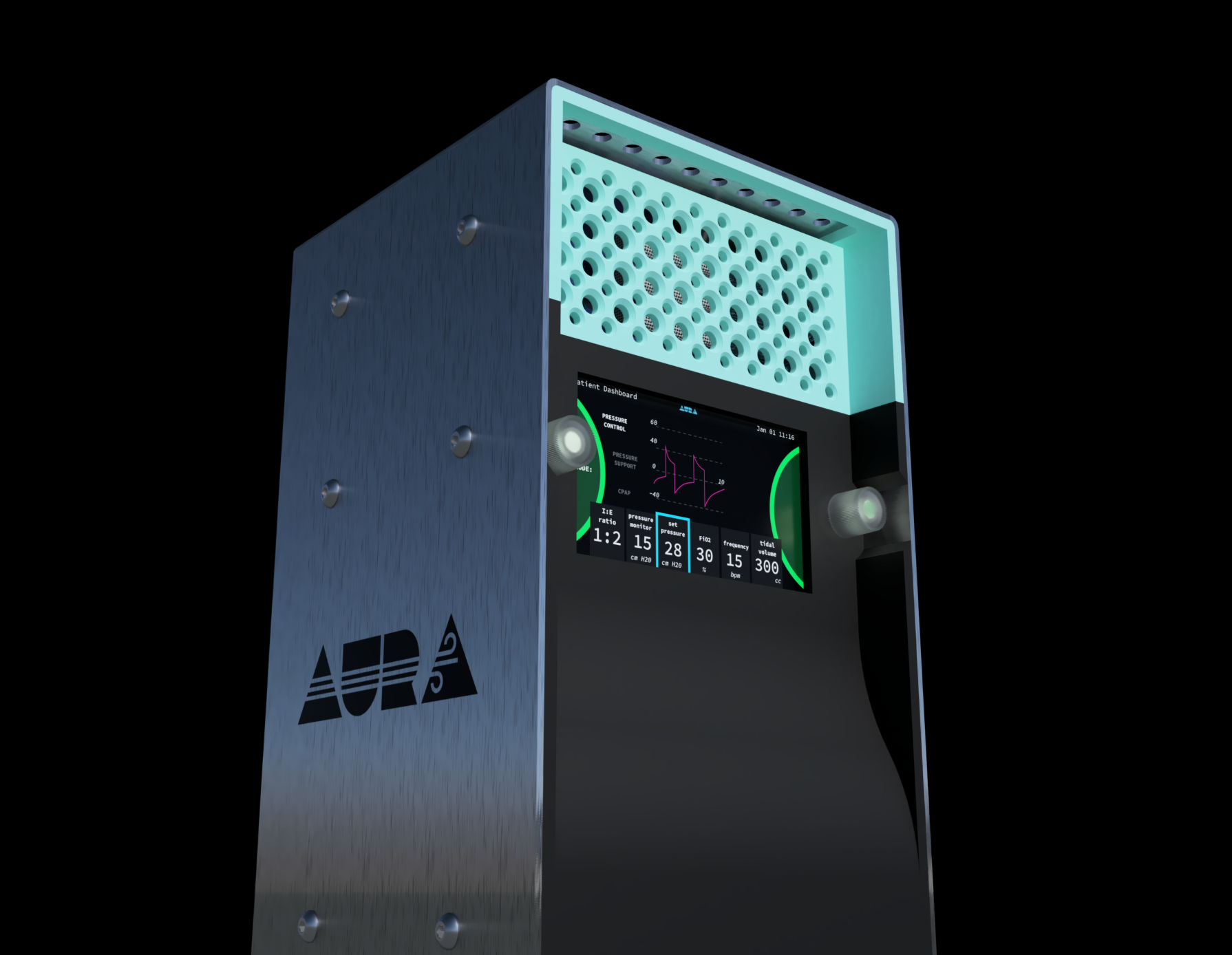
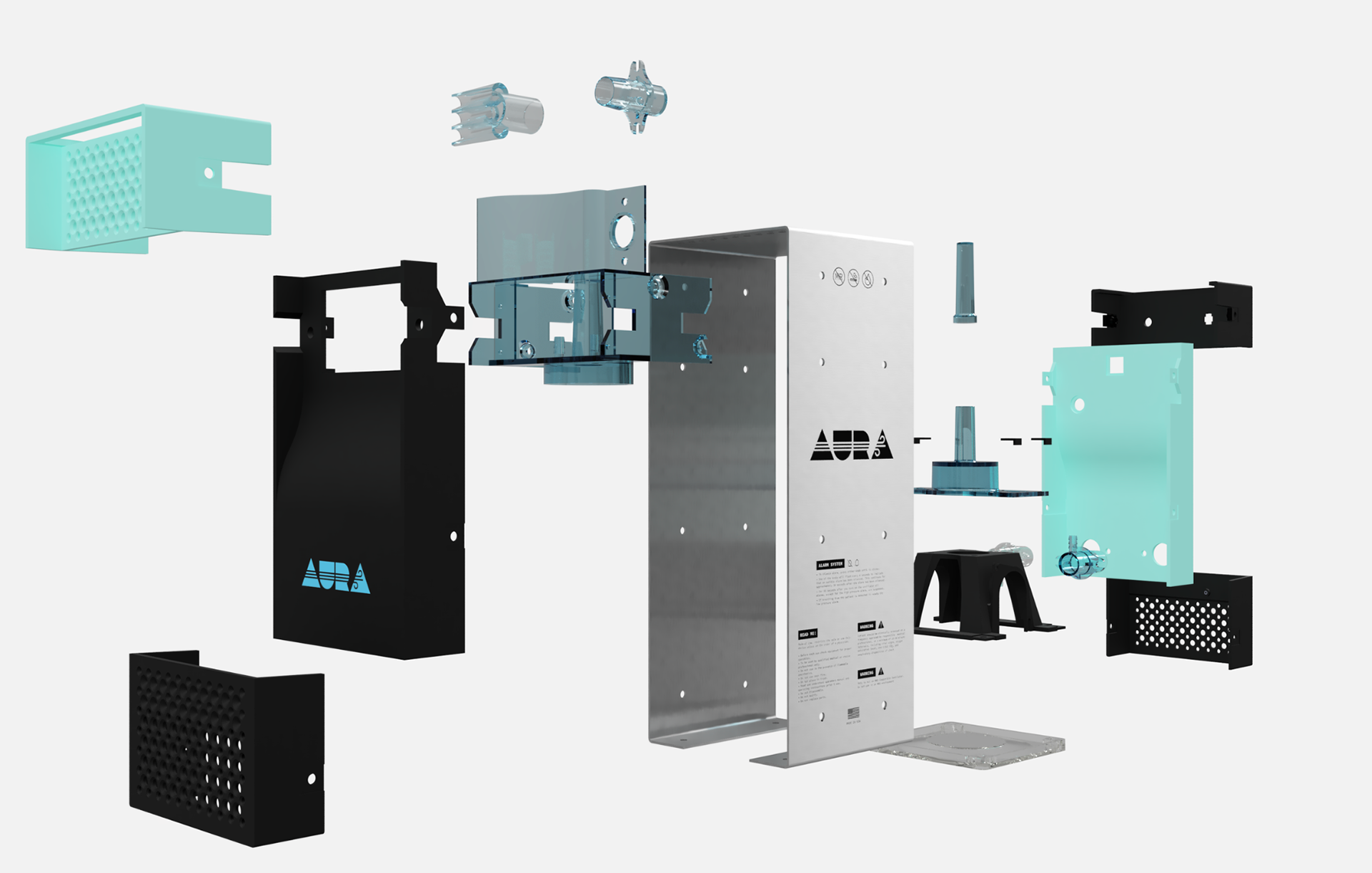
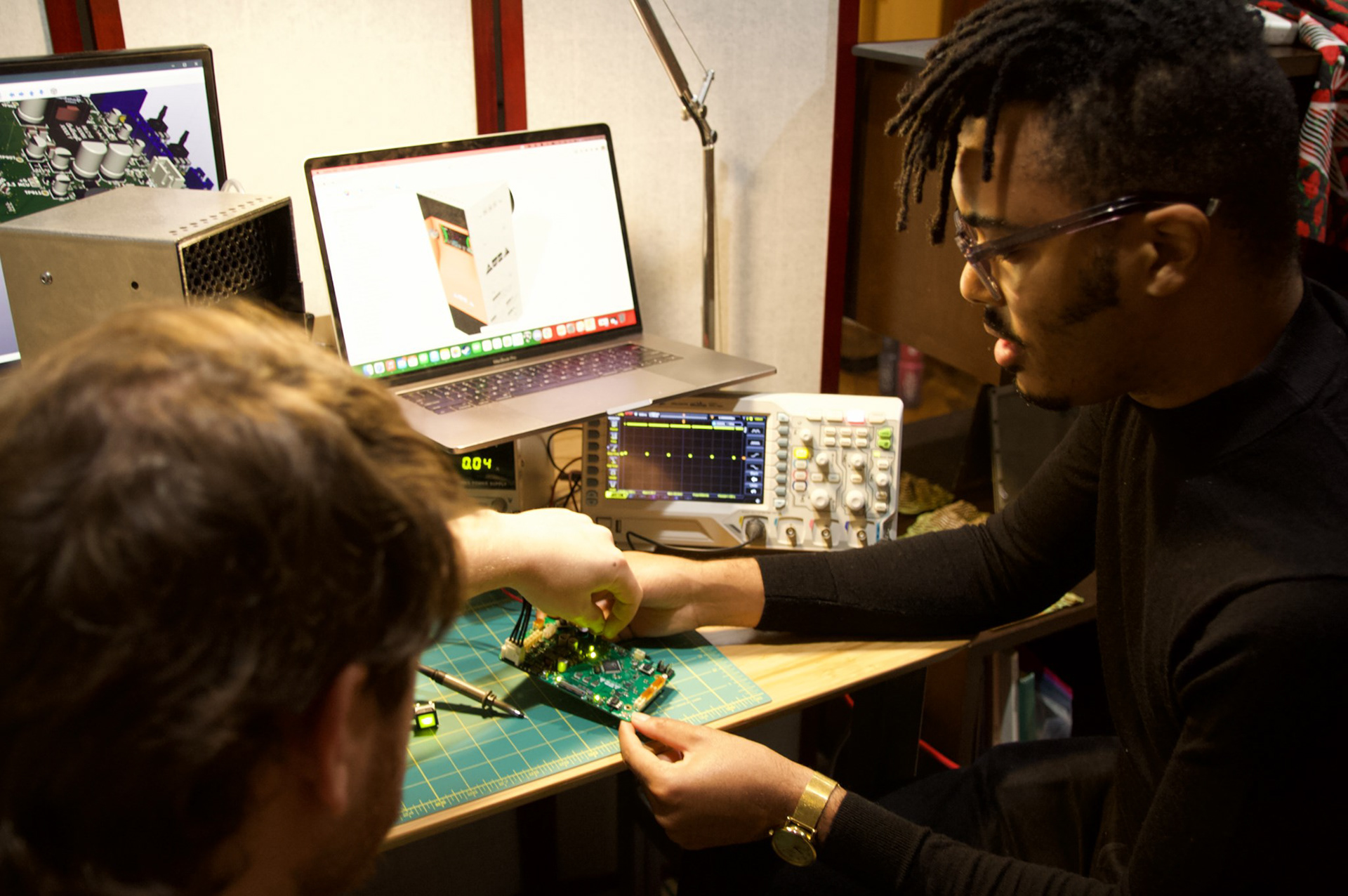
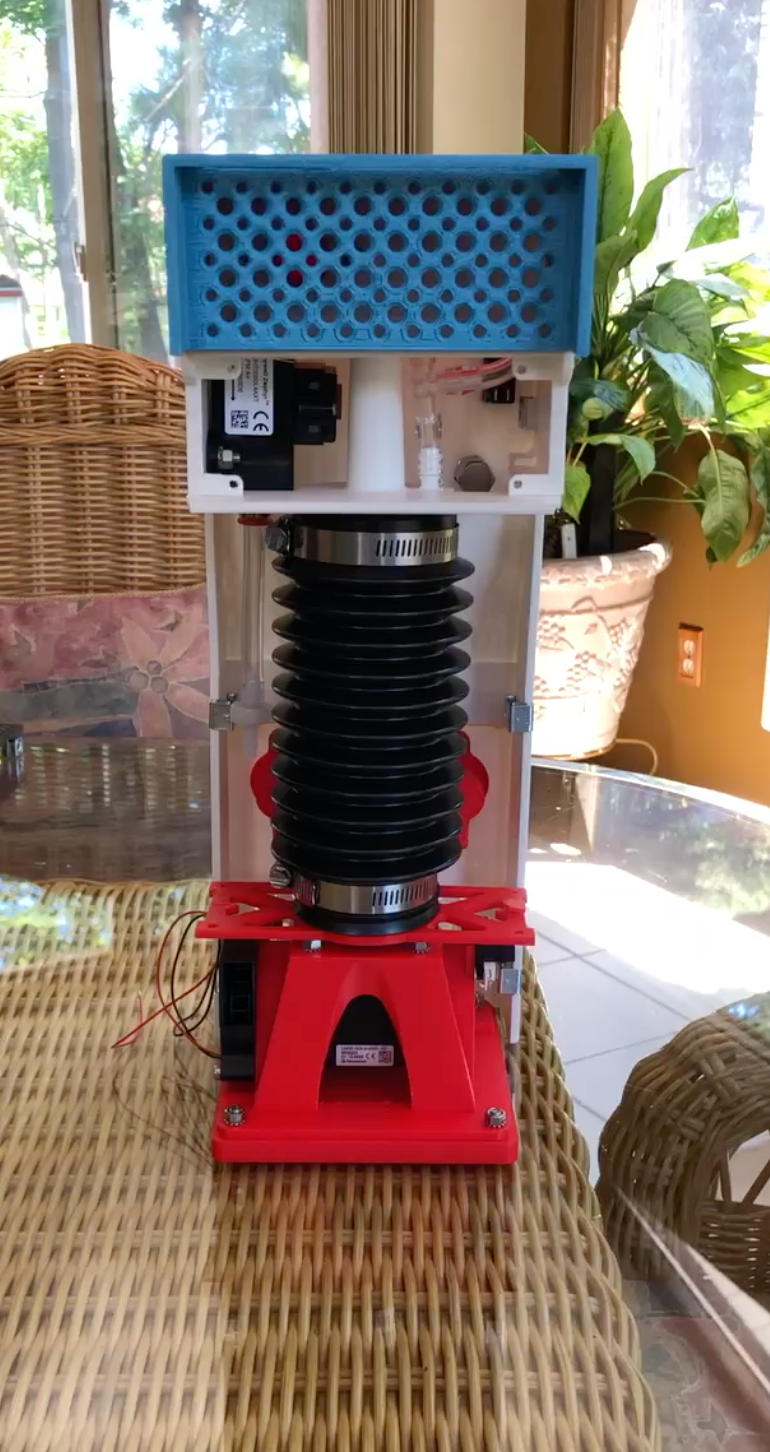
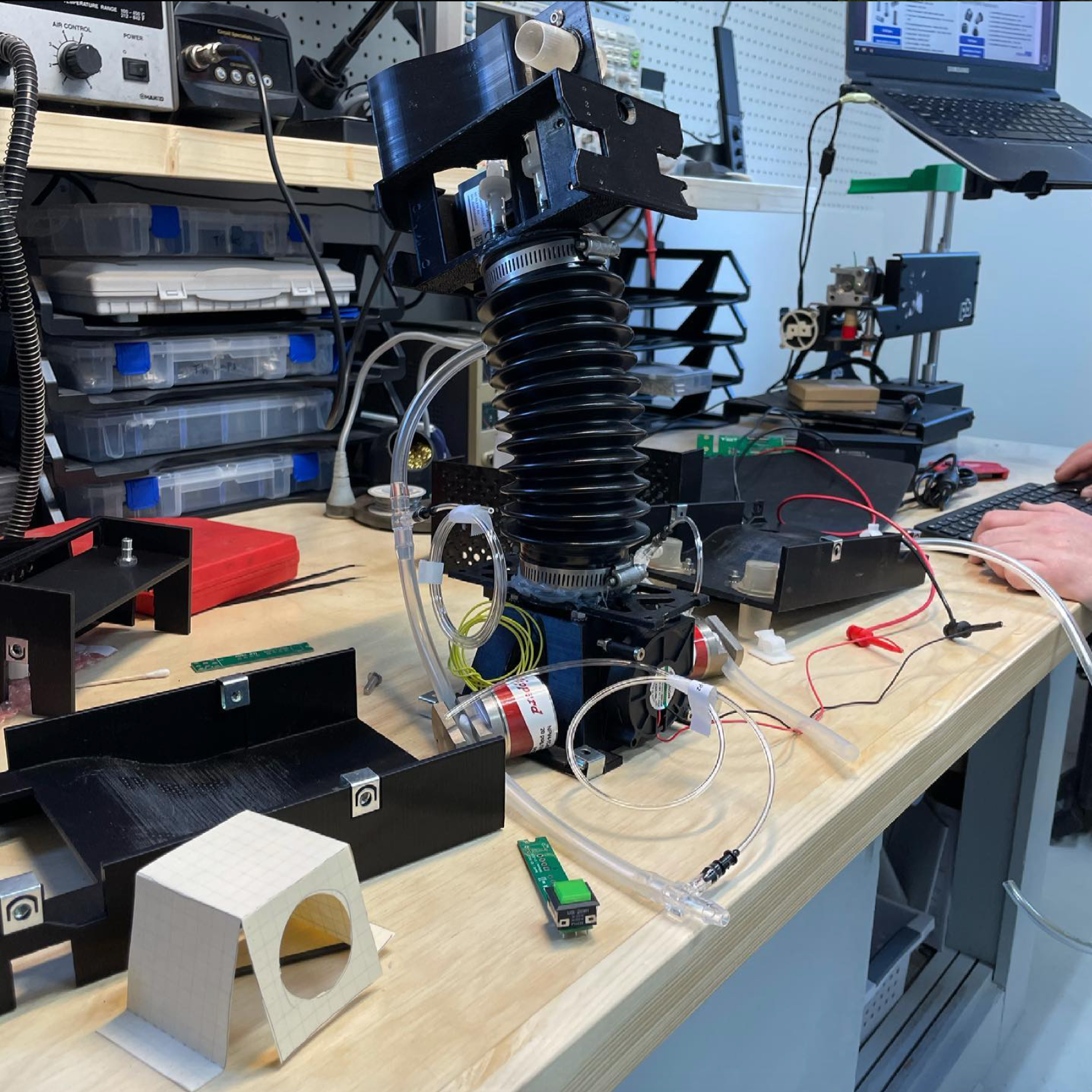
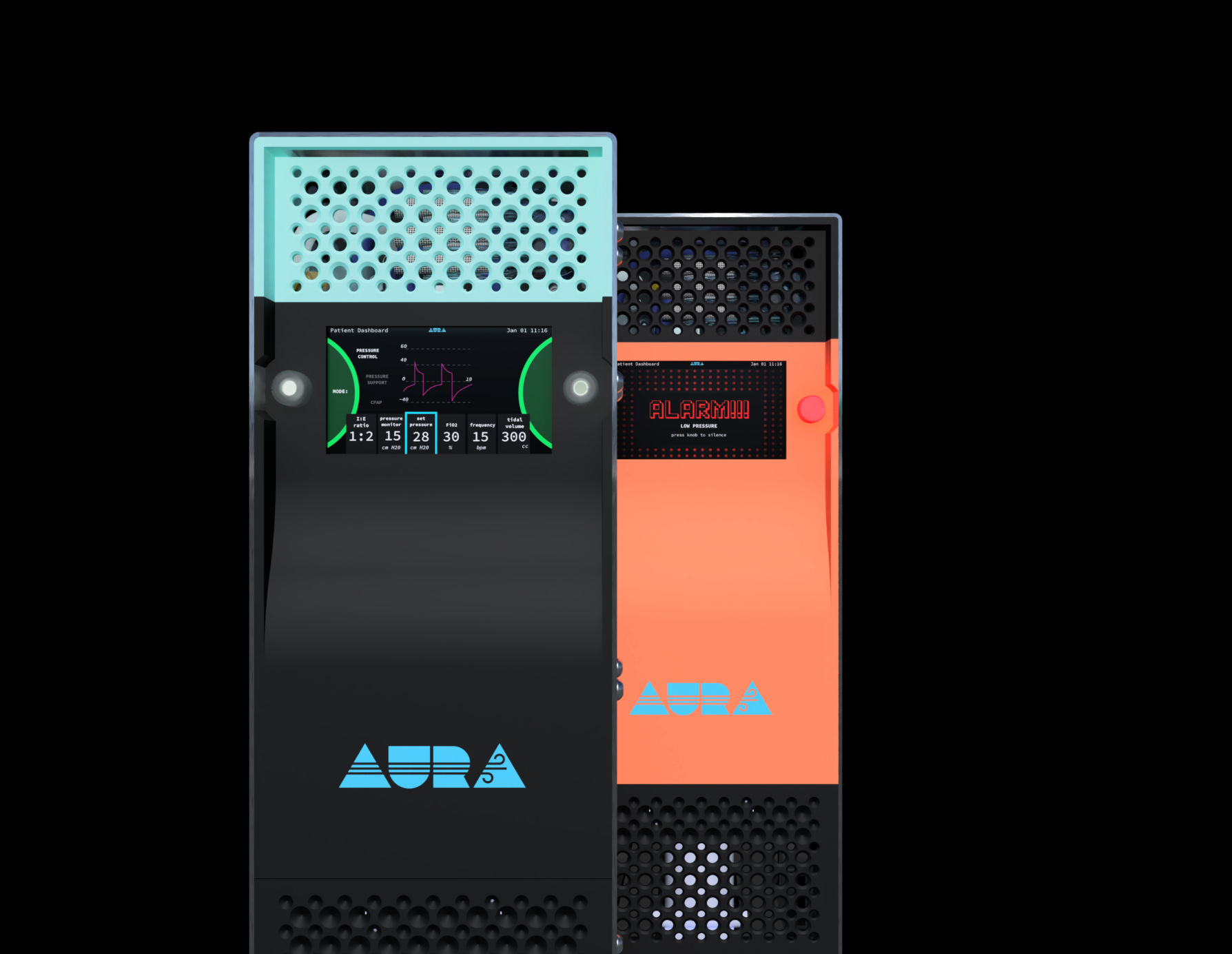
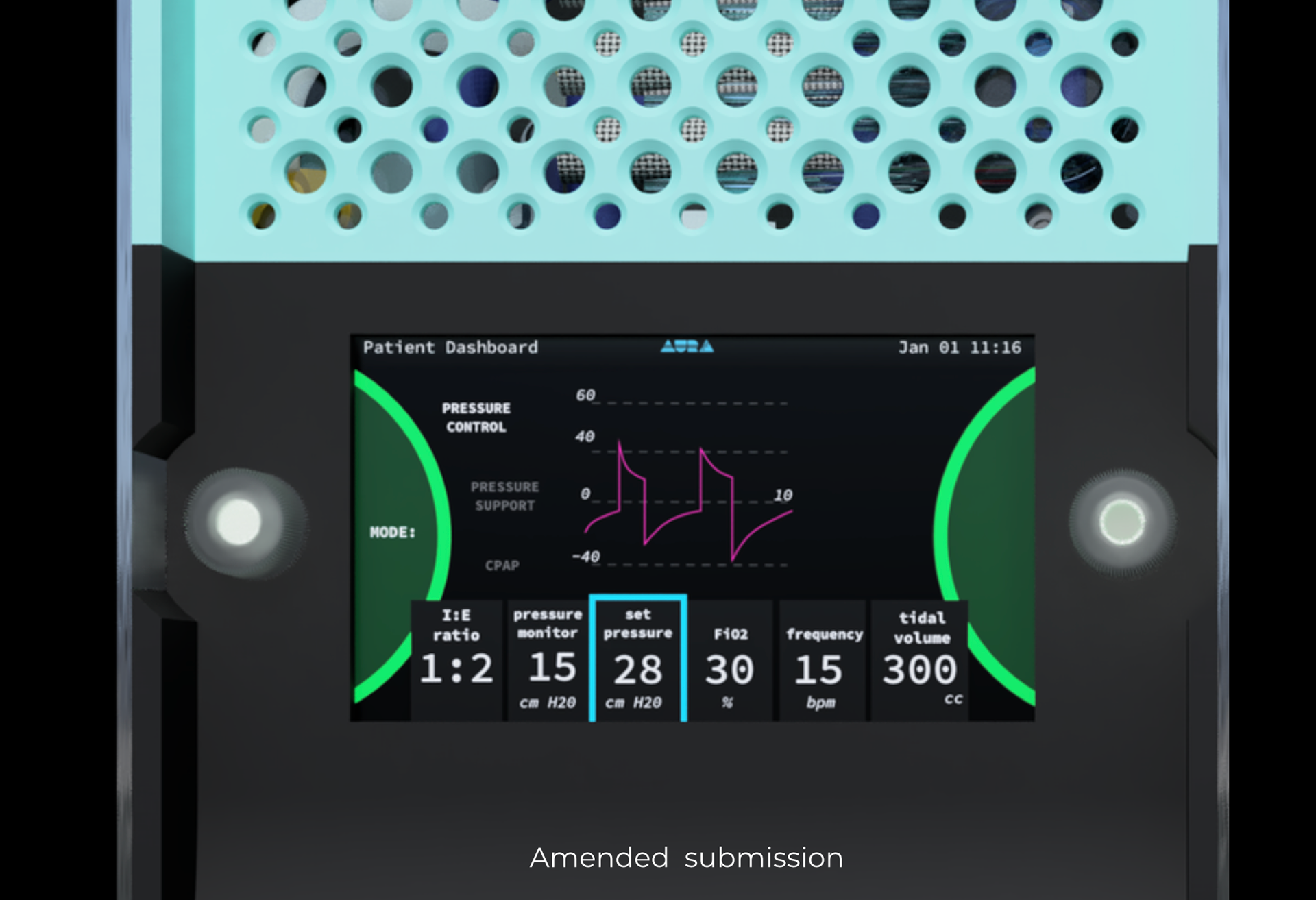
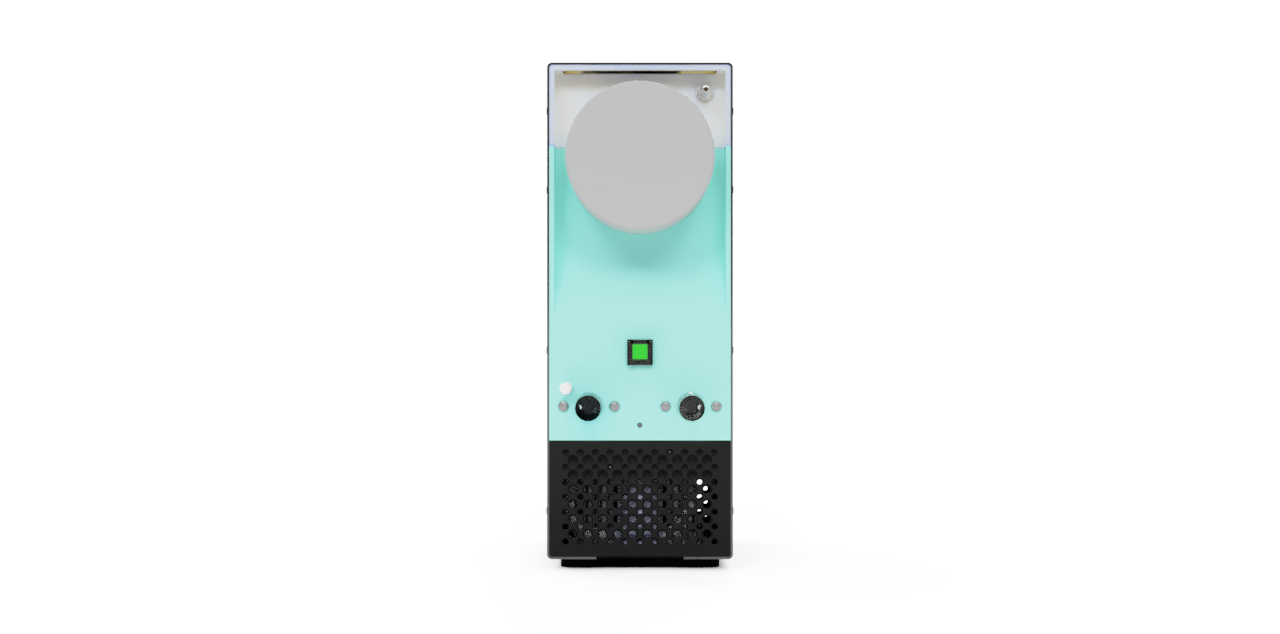
A reservoir and a new power switch were added as a part of a final FDA submission to improve performance and safety
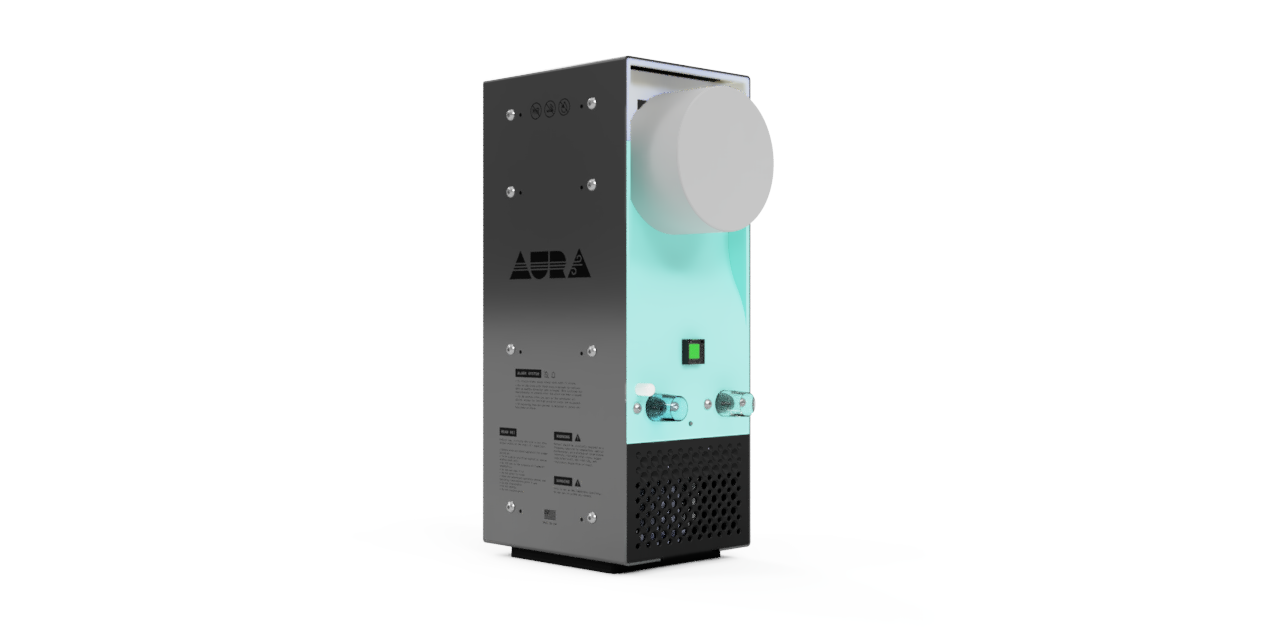
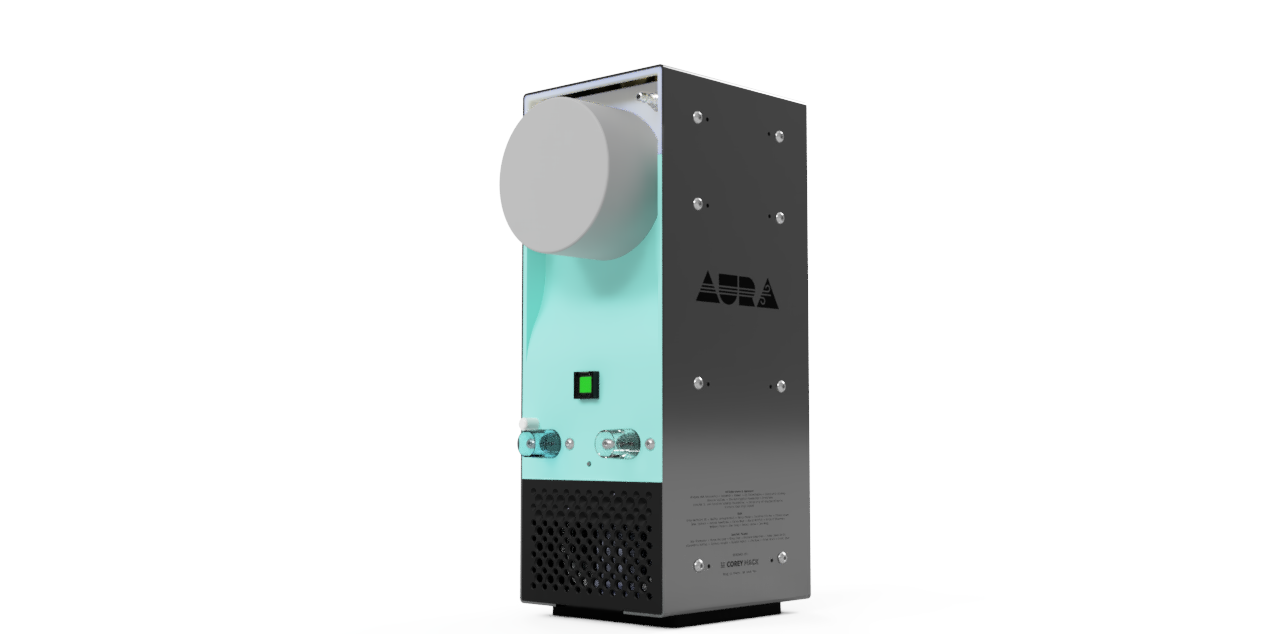
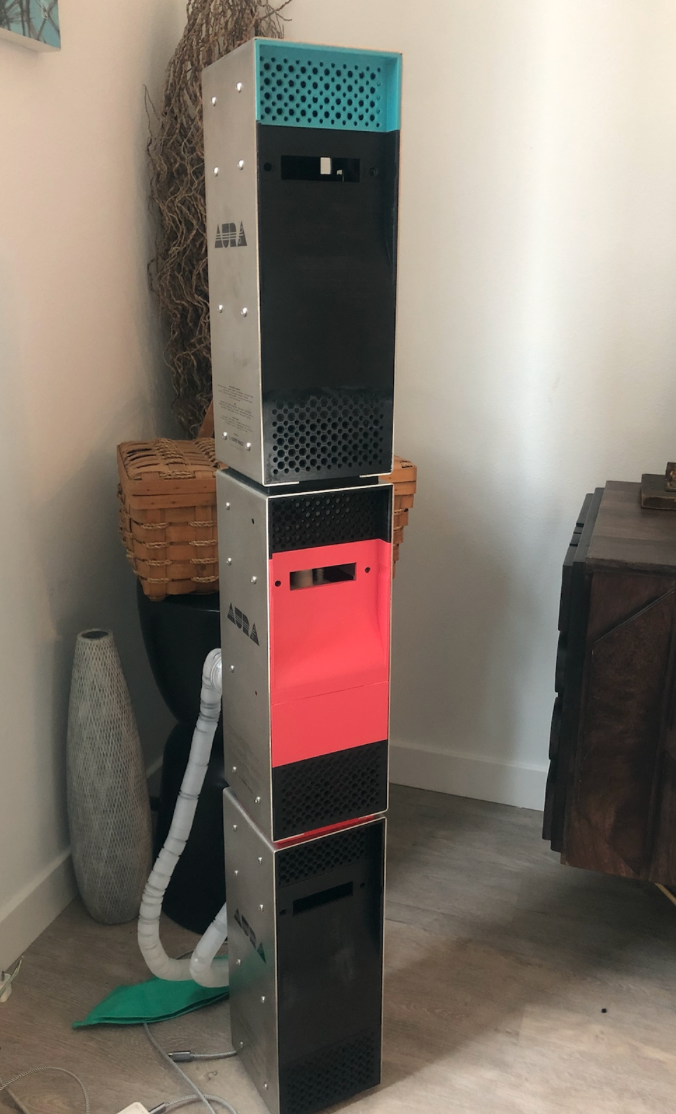